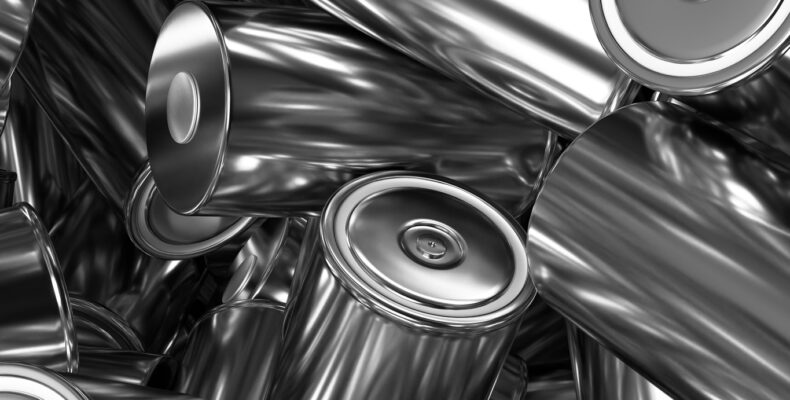
Anode battery technology: untapped potential?
As material optimisation is sought to improve peak battery performance, innovation within battery technology is vital. Such innovation is driven by schemes such as the Faraday battery challenge (part of the UK Research and Innovation Challenge Fund), where £541 million has been made available between 2017 and 2025 to encourage the growth of a strong battery sector in the UK. At the European Patent Office, 2023 saw a 28% increase in growth for patent applications relating to batteries.
The story so far
Within battery technology, the anode, cathode, and electrolyte elements are all subject to research and development in an attempt to manufacture and commercialise batteries that are sustainable, effective and affordable.
It will be appreciated that the main factors to consider when commercialising a battery material are energy density, power density, cost, and lifetime. Within the battery industry, a trade-off between these factors must be found.
According to European Patent Office data, innovation continues to be weighted towards lithium-ion batteries, with 45% of battery-related patent applications being directed to this technology, versus 7.3% for other battery chemistries, and the remainder focussing on aspects of manufacturing and engineering.
To date, most of the technological advancements in lithium-ion battery technology have been directed towards the research and subsequent implementation of novel cathode materials. These have been the focus of battery research because they are the most expensive component of the battery. Common cathode materials tend to be based on cobalt, nickel, and manganese oxides, and studies estimate that cobalt supplies will be exhausted before 2030 based on current market requirements. Therefore the search for alternative materials is vital in order to reduce the dependency on these dwindling supplies of cobalt.
The next chapter
Anode materials have not been as extensively researched, meaning there are significant opportunities to improve battery performance through anode optimisation, either alone or in combination with cathode optimisation.
Current lithium-ion battery technology mainly uses three different types of anode material: graphite, silicon and lithium titanate (LTO).
Graphite is by far the most common; it is estimated that 90-95% of lithium-ion batteries use a graphite anode. Lithium ions can be easily stored during charging, through intercalation between the graphite layers, and deintercalation occurs during discharging. Graphite displays an average energy density. This is the most affordable of the three materials and has an acceptable lifetime, of between hundreds and a couple of thousand cycles. But whilst the power density under discharge is good, there are safety limitations when using fast charging. These safety limitations can be attributed to lithium dendrite formation or lithium plating, where metallic microstructures form on the anode during the charging process, due to the inability of the anode to absorb the lithium ions at a sufficient rate. These lithium dendrites may cause internal short circuits, resulting in catastrophic battery failure.
Silicon or silicon oxide anode materials are found in higher energy density batteries. The high energy density of the silicon-based anode may be attributed to the high potential capacity to store lithium ions – each silicon atom can bind up to 3.75 lithium ions, whereas each carbon atom in graphite binds only 1/6 of a lithium ion. Silicon or silicon oxide can be mixed with graphite to achieve improved potential capacity when compared to pure graphite anodes. Silicon-based anode materials are affordable – more expensive than graphite, but notably less than LTO – and the power density is good. The main problem for silicon-based anode materials is their lifetime. Upon intercalation or deintercalation of lithium ions, the structure of the anode experiences huge volumetric fluctuations, limiting lifetime to only a couple of hundred cycles.
LTO anode materials exhibit high power and a long lifetime, which may be up to ten times longer than graphite and silicon-based batteries. After optimisation, one can reasonably expect tens of thousands of cycles, and LTO based batteries are appreciably faster to charge than other alternatives. However, there are a limited number of lithium ions that may be contained within the crystal structure, resulting in the battery size being problematically large; taking the example of use within an electric vehicle, about half the vehicle would be taken up by the battery. For the same reason, batteries implementing LTO anodes are very expensive.
Current research within the area of anode materials includes the development of hard carbon and soft carbon-based materials. These are higher voltage materials than graphite, which do not allow dendrite formation, improving the safety of the battery. Issues related to these materials include reducing side reactions and lithium loss. Mixed niobium oxide materials are also an anode material of interest, as these display the same benefits as LTO but have a more competitive energy density and reduced cost.
Potential for development
There is clearly potential for further anode development, across a wide range of uses, to overcome challenges and limitations with current battery technology.
According to the Battery 2030 report by McKinsey, the need for Li-ion batteries is expected to grow by about 27% annually, with demand increasing from about 700 GWh in 2022 to around 4.7 TWh by 2030. The Li-ion battery value chain is predicted to increase from about $85 billion in 2022 to over $400 billion in 2030.
We can therefore expect to see continued innovation and patenting activity in relation to battery technology over the coming years.
Barker Brettell has dedicated automotive and energy sector groups that can assist and advise you. If you have an invention you would like to protect, do not hesitate to contact the author or your usual Barker Brettell attorney.